- Overview
- Description
- Specifications
- Experiment & Sample preparation
- Data Analysis
- Publications
- Staff
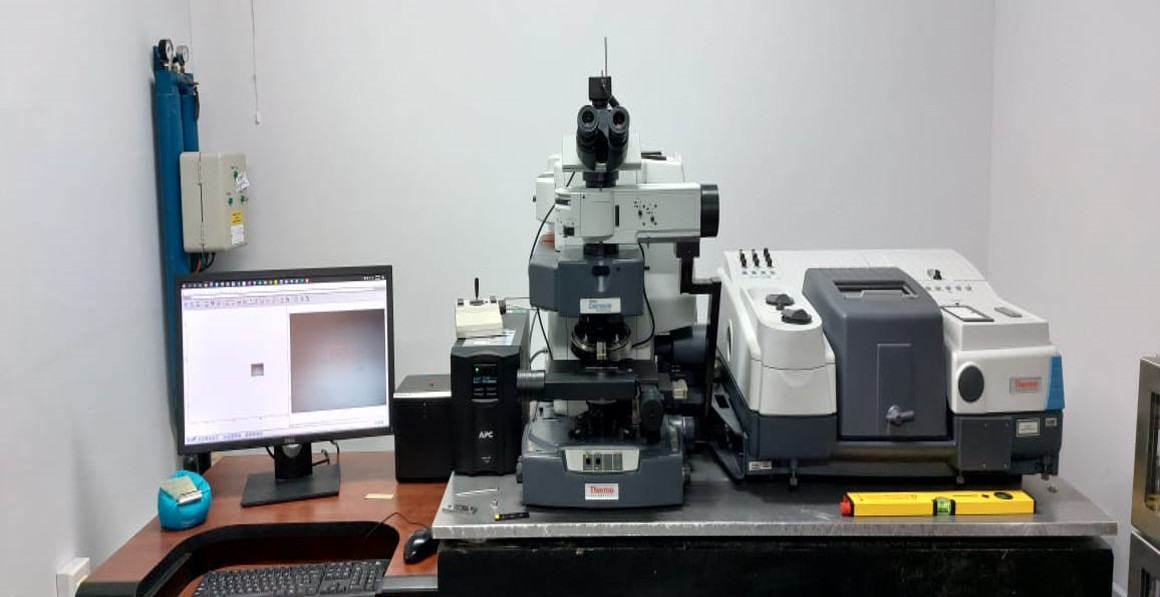
Welcome to the IR beamline at SESAME.
The IR beamline was designed and implemented in the framework of a partnership between SESAME and the SOLEIL Synchrotron facility in France. It came into operation in November 2018 to serve users of the infrared scientific community.
The beamline is located at bending magnet BM02 of SESAME’s storage ring and utilises both edge and constant field radiation ranging from 2 to 25 µm in wavelength. The end station is equipped with a FTIR (Fourier Transform Infrared) spectrometer coupled to an IR microscope that can also be used with the internal Globar lamp during machine shutdown.
FTIR interferometry is a powerful tool for a variety of research fields. It is based on the identification and imaging of IR-active vibrational modes of molecular components at microscopic scale.
The potential applications of the IR beamline cover a wide range of research fields, including life sciences, pharmaceuticals, diagnostics, environmental science, materials science, archaeology, cultural heritage, and art restoration, amongst others.
The SESAME IR beamline is currently equipped with a new endstation of the Bruker Vertex 70v FTIR spectrometer coupled to Hyperion 3000 IR-vis Microscope. The coupling with IRSR is in progress and will be commissioned in May 2022.
The IR beamline recently joined the INFN-CHNet network. For more information, click here
The beamline is also giving access to a second offline endstation (Globar IR source) equipped of 8700 Thermo Scientific FTIR spectrometer coupled with a Thermo Scientific Nicolet Continuum XL IR-microscope (Thermo Fisher Scientific®, USA).
IR microspectroscopy is a vibrational technique that is non-destructive and which is exhibiting a strong interest at synchrotron facilities. It combines the spatial resolution of a microscope together with the high chemical sensitivity of the IR spectrometer. In addition to the SR-IR source broad spectral emission and the wavelength characteristics, Infrared Synchrotron sources provide advantages in its brightness/brilliance (about 1000 times brighter) with a signal-to-noise ratio that cannot be achieved by the conventional sources. The mid-infrared region of 2.5-25 µm (4000-400 cm-1) is very informative on the microscopic scale (a few tens of micron resolution). This is mainly because most of the existing molecular groups have their vibrational energies in this spectral range. Moreover, the spatial resolution is no longer controlled by the geometrical aperture size, but rather by the numerical aperture of the optical system and the wavelength of the light. Therefore, the spot size is set to diffraction limit (~ 3 to 10 µm) in confocal geometry. An optical system used in diffraction limit geometry may use less than 1% of the light emitted from a conventional source whereas Synchrotron sources are optimally matched.
The science mission in all the Synchrotron facilities defines performance goals reaching from the mid-IR (2.5–25μm) to the Far-IR (25–1000μm) regions, SESAME IR-beamline has been designed with a maximum acceptance opening angle of 39 mrad (H) x15 mrad (V) that will utilize infrared Synchrotron emission of the two main sources of radiation; the edge radiation (ER) and the constant field of the bending magnet (BM). It has been designed to transport Infrared radiation to the experimental end station with the minimum aberrations. To ensure the optimum beam delivery, the optical set up delivering the Synchrotron radiation emitted in the infrared range from a bending magnet of the storage ring SESAME has been considered and examined using simulation software packages such as SRW and SPOT X.
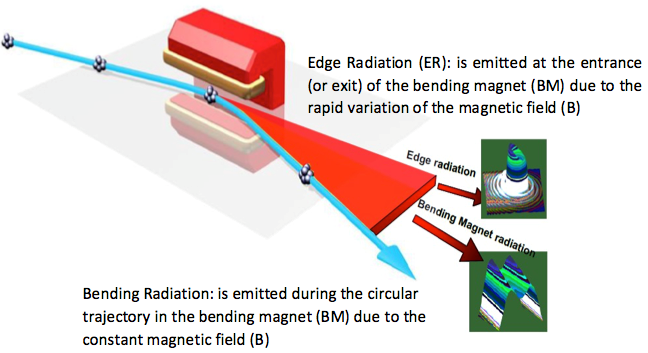
The beamline has been designed to produce a dual beam in the experimental floor, which enables serving in the future, two independent end stations. With the expected growth in user demand, and in diversity of scientific applications, a second end station will be easily implemented, without redesigning any component of the initial set up. An easy mirror swapping is planned to allow the simultaneous operation of the two modes/end stations.
Experimental Station [Instrumentation, set up and accessories]
Being designed for optimum performance from the near- to the far-IR, a suite of single and an array of detectors equip the end station to cover the whole IR range. The wide range of applications call for a versatile suite of sample environments and sampling methods coupled with off-line service for sample preparation and handling, as well as, data analysis.
Beam coupling with the FTIR spectrometer via the matching box
The coupling box, positioned after the exit of the beam from the last KBr window and before the spectrometer, allow reshaping the divergent beam, rectangle, in a more or less squared beam, collimated. This collimated beam enters the interferometer, and can be moved in X, Z direction, and tilted in order to optimize the coupling and fitting with the Schwarzschild of the microscope.
The beamline has been designed to produce a dual beam in the experimental floor, which enables serving in the future, two independent end stations. One end station will be made operational at initial development step of the beamline. With the expected growth in user demand, and in diversity of scientific applications, a second end station will be easily implemented, without redesigning any component of the initial set up. An easy mirror swapping is planned to allow the simultaneous operation of the two modes/end stations.
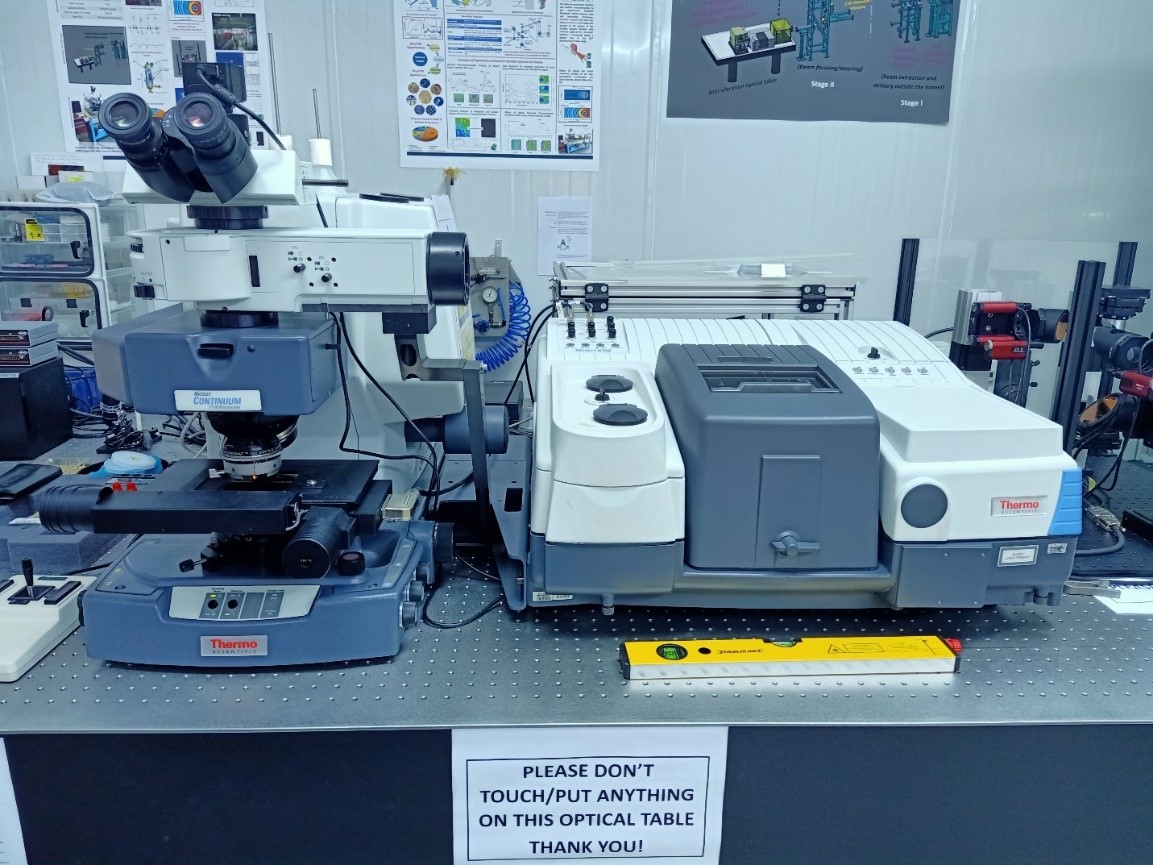
The end station encompasses the 8700 Thermo Scientific© FTIR spectrometer (equipped with CaF2, KBr, and Solid-state beam splitters, and internal DLaTGS detectors of KBr and Polyethylene Windows, in addition to an InGaAs detector for NIR). The spectrometer is coupled to a Thermo Scientific© Nicolet Continuum IR-microscope equipped with 15x and 32x for transmission/reflection, ATR, and grazing incidence angle IR objectives, together with MCT-B and small area MCT-A* detectors. The endstation microscope allows micro-spectroscopic chemical imaging studies, with DIC and fluorescence microscopy capabilities.
BM D02
Standard Endstation
-Wide Band Blue Fluorescence Cube 450-480nm
-Wide Band Green Fluorescence Cube 510-550nm
-Wide Band UV Fluorescence Cube 330-385nm
DTGS
InGaAs
Sample
Sample Holders
-Slide-on 15x Si-ATR.
-Grazing Incidence Reflectance;
-Diamond compression cell;
-KBr micro-compression cell;
-Small hydraulic press for KBr pellets (7mm);
-Monolayer Grazing Angle Specular Reflectance Accessory.
DTGS
- TE Cooled DLaTGS Detector with KBr Window (12,500-350 cm-1);
- DLaTGS Detector with Polyethylene Window (700-50 cm-1).
Detection
InGaAs
InGaAs detector for NIR (12.000-3.800 cm-1).
Detection
MCT
- 50um MCT-A Detector;
- MCT-B Detector.
Detection
The simplest way to collect infrared spectra is in the transmission mode, in this mode, thin samples are generally needed, 5 to 30 µm is the typical thicknesses for the transmission measurements, polymers, biological tissues and geological and organic materials can be studied in this mode.
The second most common technique of recording infrared spectra is the reflection mode. In reflection mode, the infrared beam penetrates the sample, then reflects off from the substrate, returns to the sample and passes back through the illumination objective, Samples studied in reflection are normally have high reflective properties, or polished samples which cannot be cut by microtome to a thin section.
Highly reflective substrates have commonly thickness of coating material approximately of 1-10µm. The flat and smooth sample surfaces are important requirements in this mode to avoid scattering. Irregular surfaces reduce the collection efficiency, and produce oscillations in the background of the spectra. In special cases, irregular surfaces can be pressed to adjust the slope of the sample with respect to the incident light, and to make the surface of the sample parallel to the microscope stage.
Attenuated total reflection is a technique that probes the near-surface region of materials. Materials that are not reflected and cannot be cut into thin sections, such as liquids, semi solids, and pliable solids, like rubber and plastic, can be analyzed using an ATR technique. This method works well with samples that are too opaque or too thick for standard transmission methods. The sample is placed in contact with a crystal of high refractive index such as ZnSe, Ge, or diamond. Total internal reflection occurs along the crystal-sample interface. The IR beam entering the crystal is totally internally reflected within the crystal. The beam creates an evanescent wave, which projects orthogonally into the sample in intimate contact with the ATR crystal. Some of the beam absorbed by the sample and the reflected radiation return to the objective.
Grazing incidence sampling in FTIR spectroscopy is a useful technique for measurement of thin films on highly reflective substrates. The technique is often used for sub-micron thick coating substrates. Films that are typically less than 1µm thick can get benefits from the technique. The basics of the technique involve measurements of the reflected beam from a sample surface at a given angle of incidence. Using Schwarzschild objective, that contains a beam mask, transmits only the grazing incidence rays onto the sample.
- Instrumentation:
IR Microscope coupled to a Fourier transform IR spectrometer bench Simultaneous fluorescence measurement, ATR and grazing incidence objectives.
Thermo Fisher Optics - Nicolet Continuum: Köhler Illumination for Reflection and Transmission, Brightfield, Darkfield and Polarized Light, Objectives: 15x (0.58 NA), 32x (0.65 NA) installed. The microscope is equipped with DIC (Differential Interference Contrast) Optics; Fluorescence illumination of: -Wide Band Blue Fluorescence Cube 450-480nm, -Wide Band Green Fluorescence Cube 510-550nm, -Wide Band UV Fluorescence Cube 330-385nm.
- Possible techniques of FTIR spectroscopy/microscopy include:
- ATR Spectromicroscopy
- Grazing incidence angle
- Fluorescence illumination:
- Wide Band Blue Fluorescence Cube 450-480nm
- Wide Band Green Fluorescence Cube 510-550nm
- Wide Band UV Fluorescence Cube 330-385nm
- Reflection
- Transmission
- Sample holders:
Different mounting accessories for different types of samples.
- Liquid transmission demountable cell - Spacers of various thicknesses used to vary the cell pathlength (6µm-1000µm);
- Slide-on 15x Si-ATR.
- Grazing Incidence Reflectance;
- Diamond compression cell;
- KBr micro-compression cell;
- Small hydraulic press for KBr pellets (7mm);
- Monolayer Grazing Angle Specular Reflectance Accessory.
- Detectors
- TE Cooled DLaTGS Detector with KBr Window (12,500-350 cm-1);
- DLaTGS Detector with Polyethylene Window (700-50 cm-1);
- Room Temperature InGaAs Detector for NIR (12.000-3.800 cm-1)
- 50um MCT-A Detector (11.400-700 cm-1)
- MCT-B Detector (11.700-450 cm-1)
- Equipment Available on- Site
- Optical Stereomicroscope.
- Basic tools for sample manipulation.
- Polarization-Modulation Infrared accessory with a universal TOM for IRRAS, VLD, and DIRLD.
- Control Software
- Beamline EPICS, Endstation OMNIC® 9.2.41, OMNIC Atlus® 9.1.24. Proprietary software (Copyright © 1992-2012 Thermo Fisher Scientific Inc.).
- Data Output Type
- Transmittance/Reflectance/Absorbance IR single spectra, interferograms, visible images, and hyperspectral images/maps.
- Data Output Format
- OMNIC® (Copyright © 1992-2012 Thermo Fisher Scientific Inc.) [*.spa and *.map] (with possible conversion to other formats.)
- Data Analysis Software
- On-site and remote access to OMNIC® 9.2.41, OMNICMC® v 9.1.0, OMNIC Atlus® 9.1.24. (Copyright © 1992-2012 Thermo Fisher Scientific Inc.). The Unscrambler X® v 10.5. (Copyright © CAMO Analytics). CytoSpec® v 1.3.02. (Copyright © 2000-2020 Peter Lasch). Quasar®.
2025 (2), 2024 (3), 2023 (2), 2022 (7), 2021 (6), 2020 (2), 2017 (2), 2016 (3), 2012 (1), All (28)
2025
- Insights into the anticancer and anti-inflammatory activities of curcumin-loaded quercetin nanoparticles: in vitro bioassays coupled with synchrotron infrared microspectroscopy
Mater. Adv., Vol. , pp. (2025)
S Sunoqrot, S Abusulieh, LA Dahabiyeh
doi: 10.1039/D4MA01202J - Confirmation of L-phenylalanine's toxic fibrillary formation and its modulation by D-phenylalanine at different ratios and pH values by using synchrotron FTIR
Spectrochimica Acta - Part A: Molecular and Biomolecular Spectroscopy, Vol. 333, pp. (2025)
S. K. Hacıosmanoglu, M. G. Akkurt, E. Atas, G. Kamel, S. Uyaver, M. Kazanci
doi: 10.1016/j.saa.2025.125891
- Biochemical analysis of Hyalomma dromedarii salivary glands and gut tissues using SR-FTIR micro-spectroscopy
Scientific Reports, Vol. 14 - 1, pp. 8515 (2024)
S.H.M. Hendawy, H.F. Alzan, H.S.M. Abdel-Ghany, C.E. Suarez, G. Kamel
doi: 10.1038/s41598-024-59165-6 - Green plastics: Direct production from grocery wastes to bioplastics and structural characterization by using synchrotron FTIR
Spectrochimica Acta Part A: Molecular and Biomolecular Spectroscopy, Vol. 323, pp. 124919 (2024)
O Aras, G Kamel, M Kazanci
doi: 10.1016/j.saa.2024.124919 - A Comprehensive Study on How Different Mineralization Processes Affect Native Structure of Wetspun Collagen Fibers and Mineral Quality by Using S(Ftir)
Elsevier SSRN (Social Science Research Network), Vol. , pp. (2024)
K. Selcuk Haciosmanoglu, A. Belet, G. Kamel, M. Kazanci
doi: 10.2139/ssrn.4775064
- Synchrotron Fourier Transform Infrared Microspectroscopy (Sftirm) Analysis of Beta Amyloid Aggregation/Clearance in Al-Induced Alzheimer'S' Disease in Rat Brain Hippocampal Tissue
Elsevier SSRN (Social Science Research Network), Vol. , pp. (2023)
S. K. H. Khalil, W. El hotaby, G. Abdel-Raouf Ahmed, G. Kamel, H. H. A. Sherif, L. Abbas
doi: 10.2139/ssrn.4560767 - IR microspectroscopic investigation of the interaction of some losartan salts with human stratum corneum protein and its effect on losartan transdermal permeation
PLOS ONE, Vol. 18 - 6, pp. e0287267 (2023)
R.S.H. Mansour, A.Y. Al Khawaja, I.I. Hamdan, E.A. Khalil
doi: 10.1371/journal.pone.0287267
- Synchrotron radiation infrared microspectroscopy: Insights on biomedicine
Applied Spectroscopy Reviews, Vol. , pp. 1-20 (2022)
A. Refaat, G. Kamel
doi: 10.1080/05704928.2022.2052308 - Synchrotron Fourier-transform infrared microspectroscopy: Characterization of tumor infiltrating monocytes stimulated by the secretome of inflammatory and non-inflammatory breast cancer cells,
Conference: AACR Annual Meeting 2022,
New Orleans (US) 18/11/2021 (2022)
A. El-Sharkawy, G. Kamel, H. Mohamed, M. El-Shinawi, M. Mohamed, N. El-Husseiny
doi: 10.1158/1538-7445.AM2022-6132 - Plasma drop and thin-film revealed distinguished molecular structure in pre-eclampsia: An investigation using synchrotron Fourier-transform infrared microspectroscopy
Journal of Pharmaceutical and Biomedical Analysis, Vol. 220, pp. 114981 (2022)
L.A. Dahabiyeh, R.S.H. Mansour, W. Darwish, S.S. Saleh, G. Kamel
doi: 10.1016/j.jpba.2022.114981 - Synchrotron Fourier-Transform Infrared Microspectroscopy: Characterization of in vitro polarized tumor-associated macrophages stimulated by the secretome of inflammatory and non-inflammatory breast cancer cells
Biochimica et Biophysica Acta - Molecular Cell Research, Vol. , pp. (2022)
H. Mohamed, G. Kamel, N. El-Husseiny, A. El-Sharkawy, A. El-Sherif, M. El-Shinawi, M. Mohamed
doi: 10.1016/j.bbamcr.2022.119367 - Synchrotron-Radiation-Based Fourier Transform Infrared Microspectroscopy as a Tool for the Differentiation between Staphylococcal Small Colony Variants
Antibiotics, Vol. 11 - 11, pp. (2022)
AG Al-Bakri, LA Dahabiyeh, E Khalil, D Jaber, G Kamel, N Schleimer, C Kohler, K Becker
doi: 10.3390/antibiotics11111607 - Investigating the molecular structure of plasma in type 2 diabetes mellitus and diabetic nephropathy by synchrotron Fourier-transform infrared microspectroscopy
Spectrochimica Acta Part A: Molecular and Biomolecular Spectroscopy, Vol. 264, pp. 120259 (2022)
R. Nimer, G. Kamel, MA. Obeidat, LA. Dahabiyeh
doi: 10.1016/j.saa.2021.120259 - Synchrotron Radiation Fourier Transform Infrared (SR-FTIR) spectroscopy in exploring ancient human hair from Roman period Juliopolis: Preservation status and alterations of organic compounds
Spectrochimica Acta Part A: Molecular and Biomolecular Spectroscopy, Vol. , pp. 121026 (2022)
K.O. Lorentz, G. Kamel, S.AM. Lemmers, Y. Miyauchi, E. Cubukcu, A. Alpagut, AM. Buyukkarakaya
doi: 10.1016/j.saa.2022.121026
- Characterization of Insulin Mucoadhesive Buccal Films: Spectroscopic Analysis and In Vivo Evaluation
Symmetry, Vol. 13 - 1, pp. 1-17 (2021)
M Diab, A Sallam, I Hamdan, R Mansour, R Hussain, G Siligardi, N Qinna, E Khalil
doi: 10.3390/sym13010088 - Investigation of ancient teeth using Raman spectroscopy and synchrotron radiation Fourier-transform infrared (SR-muFTIR): mapping and novel method of dating
Digest Journal of Nanomaterials and Biostructures, Vol. 16, pp. 713-724 (2021)
W. Sekhaneh, Y. Akkam, G. Kamel, A. Drabee, J. Popp
doi: 10.15251/djnb.2021.162.713 - Transcriptional modulations induced by proton irradiation in mice skin in function of adsorbed dose and distance
Journal of Radiation Research and Applied Sciences, Vol. 14 - 1, pp. 260-270 (2021)
V. Licursi, W. Wang, E. Di Nisio, F.P. Cammarata, R. Acquaviva, G. Russo, L. Manti, M. Cestelli Guidi, E. Fratini, G. Kamel, R. Amendola, P. Pisciotta, R. Negri
doi: 10.1080/16878507.2021.1949675 - Mummified Embalmed Head Skin: SR-FTIR Microspectroscopic Exploration
Spectrochimica Acta Part A: Molecular and Biomolecular Spectroscopy, Vol. , pp. 120073 (2021)
D. Moissidou, H. Derricott, G. Kamel
doi: 10.1016/j.saa.2021.120073 - The first infrared beamline at the Middle East SESAME synchrotron facility.
Journal of Synchrotron Radiation, Vol. 28 - 6, pp. (2021)
G. Kamel, S. Lefrancois, T. Moreno, M. Al-Najdawi, Y. Momani, A. Abbadi, G. Paolucci, P. Dumas
doi: 10.1107/S1600577521008778 - Synchrotron Fourier transform infrared microspectroscopy (sFTIRM) analysis of unfolding behavior of electrospun collagen nanofibers
Spectrochimica Acta - Part A: Molecular and Biomolecular Spectroscopy, Vol. 251, pp. (2021)
M. Kazanci, K. Selcuk Haciosmanoglu, G. Kamel
doi: 10.1016/j.saa.2020.119420
- Investigating the molecular structure of placenta and plasma in pre-eclampsia by infrared microspectroscopy
Journal of Pharmaceutical and Biomedical Analysis, Vol. 184, pp. 113186 (2020)
L.A. Dahabiyeh, R.S.H. Mansour, S.S. Saleh, G. Kamel
doi: 10.1016/j.jpba.2020.113186 - Synchrotron Fourier transform infrared microspectroscopy (sFTIRM) analysis of Al-induced Alzheimer's disease in rat brain cortical tissue
Spectrochimica Acta Part A: Molecular and Biomolecular Spectroscopy, Vol. 239, pp. 118421 (2020)
G.A. Ahmed, W. El Hotaby, L. Abbas, H.H.A. Sherif, G. Kamel, S.KH. Khalil
doi: 10.1016/j.saa.2020.118421
- EMIRA: The Infrared Synchrotron Radiation Beamline at SESAME
Synchrotron Radiation News, Vol. 30 - 4, pp. 8-10 (2017)
G. Kamel, S. Lefrancois, M. Al-Najdawi, T. Abu-Hanieh, I. Saleh, Y. Momani, P. Dumas
doi: 10.1080/08940886.2017.1338415 - Elucidation of penetration enhancement mechanism of Emu oil using FTIR microspectroscopy at EMIRA laboratory of SESAME synchrotron
Spectrochimica Acta Part A: Molecular and Biomolecular Spectroscopy, Vol. 185, pp. 1-10 (2017)
R.S.H. Mansour, A.A. Sallam, I.I. Hamdan, E.A. Khalil, I. Yousef
doi: 10.1016/j.saa.2017.05.026
- Optical and Micro-FTIR mapping: A new approach for structural evaluation of V2O5-lithium fluoroborate glasses
Materials Design, Vol. 89, pp. 568-572 (2016)
A.M. Abdelghany, H.A. ElBatal
doi: 10.1016/j.matdes.2015.09.159 - Monosialoganglioside-GM1 triggers binding of the amyloid-protein salmon calcitonin to a Langmuir membrane model mimicking the occurrence of lipid-rafts
Biochemistry and Biophysics Reports, Vol. 8, pp. 365-375 (2016)
M. Diociaiuti, C. Giordani, G. Kamel, F. Brasili, S. Sennato, C. Bombelli, K. Meneses, M. Giraldo, F. Bordi
doi: 10.1016/j.bbrep.2016.10.005 - Study of the biochemical effects induced by X-ray irradiations in combination with gadolinium nanoparticles in F98 glioma cells: first FTIR studies at the Emira laboratory of the SESAME synchrotron
Analyst, Vol. 141 - 7, pp. 2238-2249 (2016)
I. Yousef, O. Seksek, S. Gil, Y. Prezado, J. Sule-Suso, I. Martinez-Rovira
doi: 10.1039/C5AN02378E
- Simulation and design of an infrared beamline for SESAME (Synchrotron-Light for Experimental Science and Applications in the Middle East)
Nuclear Instruments and Methods in Physics Research Section A: Accelerators, Spectrometers, Detectors and Associated Equipment, Vol. 673, pp. 73-81 (2012)
I. Yousef, S. Lefrancois, T. Moreno, H. Hoorani, F. Makahleh, A. Nadji, P. Dumas
doi: 10.1016/j.nima.2011.12.012
Gihan KAMEL
IR Beamline Principal Scientist
Email: gihan.kamel@sesame.org.jo
Work Tel: +962 5 351 1348 (Ext. 240)